Which methane GWP value do I use? And which value should not be used?
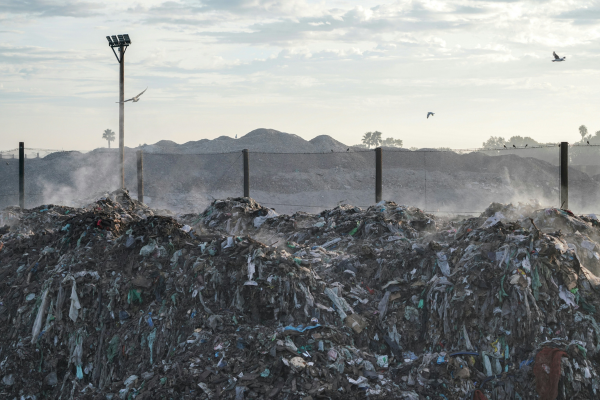
Carbon dioxide (CO2) is the primary greenhouse gas (GHG) causing long-term atmospheric emissions and climate change and therefore is a primary target for mitigation action. Methane (CH4) and other non-CO2 GHGs (e.g., nitrous oxide, sulfur hexafluoride) also warrant mitigation attention due to their potent ability to absorb terrestrial radiation. Methane, in particular, is different from most other GHGs in that it has a shorter atmospheric lifetime[1]—approximately 12 years on average – and over this shorter lifetime, it still has a significant warming effect (IPCC, 2021). All GHGs are evaluated scientifically to determine their warming effects over time. The relative effects of each GHG are reflected through a Global Warming Potential (GWP) value. But methane is a complicated GHG, as it now has multiple different published GWP values.
What is a GWP?
Global Warming Potentials (GWPs) are a quantified measure of the globally averaged relative radiative forcing impacts of particular greenhouse gases in the atmosphere. It is defined as the cumulative radiative forcing – both direct and indirect effects – integrated over time from the emission of a unit mass of gas relative to some reference gas (IPCC, 1996). Carbon dioxide (CO2) was chosen by the IPCC as this reference gas and its GWP is set equal to one (1). GWP values allow you to compare the impacts of emissions and emission reductions/removals of different gases. To be clear, GWP values are applied to units of mass (e.g., kilograms, pounds, metric tons, etc.), not to units of volume (e.g., cubic meters, cubic feet, liters).
Reference: https://ghginstitute.org/2010/06/28/what-is-a-global-warming-potential/
As you know, we use GWP values to convert the various GHGs to a common unit of CO2-equivalents (CO2-eq or CO2e) for physical GHG accounting. The normative version of GWP values is the one that integrates the warming of each gas over the conventional 100-year time horizon.[2] For methane, its atmospheric lifetime is approximately 12 years, which is much shorter than 100 years. So then, do we just ignore the other 88 years of the time horizon? As long-time readers of this blog will know, what happens to methane at the end of its atmospheric lifetime introduces a complication into how we estimate total CO2-eq emissions. At the end of each methane molecule’s atmospheric , the carbon in it is (for the most part) oxidized to CO2, which then stays in the atmosphere as CO2 for another century or more. So really, when methane is emitted, we get a radiative forcing effect double whammy—first from the methane itself followed by the CO2 that results from atmospheric oxidization.
In response to this fact, more recent IPCC assessment reports have introduced multiple 100-year methane GWP values that vary depending on the anthropogenic source of the emitted methane. For example, if methane originates from processes involving fossil fuels, then it results in an addition of CO2 to the atmosphere. In contrast, if methane originates from a biogenic source, then it just returns CO2 that was previously in the atmosphere. Unfortunately, selecting the appropriate methane GWP value is not as simple as differentiating between fossil and biogenic methane sources. In our work preparing GHG emission inventories and avoided emissions calculations it is up to us, as GHG experts, to understand which GWP value is appropriate for a given estimation. This means selecting the correct methane GWP for each emission source individually.
Before proceeding, we apologize in advance, as the following explanation on the selection of appropriate methane GWP value will require some hard thinking. Buckle up…here we go!
IPCC’s methane GWP extravaganza
At the time of writing this blog, the most up-to-date GWP values are those in the 2021 sixth assessment report (AR6) from the Intergovernmental Panel on Climate Change (IPCC), which provides three different GWP values for methane and six different labels to describe methane of different origins. To add further complication, the IPCC reports spread these mentions of methane across three different sections and across two working groups’ reports of AR6 (IPCC, 2021; IPCC, 2022). So, yes, the selection of an appropriate GWP value for the calculation of carbon dioxide equivalent (CO2-eq) of methane (CH4) emissions is even more challenging than you probably realized. But, we will explain it so you can develop the expertise to choose the appropriate values and better interpret future changes to these values by the IPCC.
Table 1: Various IPCC AR6 methane GWP values, labels, and references
IPCC AR6 GHG Labels | GWP value (100 yr) | References |
---|---|---|
CH4-fossil CH4 (fossil – fugitive and process) | 29.8 | AR6 WGI Chapter 7, Table 7.15 AR6 WGIII, Annex II, Table 9 |
CH4-non fossil CH4 (biogenic) CH4 (fossil-combustion) | 27.0 | AR6 WGI Chapter 7, Table 7.15 AR6 WGIII, Annex II, Table 9 AR6 WGIII, Annex II, Table 9 |
Methane | 27.9 | AR6 Supplemental Material for WGI Chapter 7, Table 7.SM.6 |
Note: If you want to see all three tables from AR6, click here.
Here comes some atmospheric chemistry
So, what is the difference between these three methane GWP values? We will start with the 27.9 value, as this one is determined in a more simple manner in keeping with the GWP of most other GHGs.
The 27.9 GWP value
The 27.9 GWP value, identified in the IPCC AR6 as “methane”, represents solely the radiative forcing effect of methane, relative to CO2, over the 100-year integration time.[3] This GWP value does not consider the oxidation of CH4 at the end of its atmospheric lifetime to CO2 nor the subsequent radiative forcing effect of that carbon dioxide. In other words, this “solely methane” value integrates the radiative forcing of only CH4 molecules over 100 years with no adjustments.
Figure 1: Highly simplified representation of CH4 radiative forcing over 100 years. Note: this graph does not accurately depict the decay curve of an annual methane pulse[4] over time, it depicts radiative forcing over time for CH4 released in year 0.
The 29.8 GWP value
The 29.8 GWP value, identified in the IPCC AR6 as fossil and fossil – fugitive and process, is calculated by increasing the “solely methane” value (27.9) to reflect the subsequent radiative forcing effect from CO2 added to the atmosphere through the oxidation of methane.[5] However, it is not so simple, as the CO2 from the oxidation of methane is formed at the end of a methane molecule’s atmospheric lifetime (12 years on average). Therefore, the IPCC effectively integrates the radiative forcing effect of this CO2 over, on average, approximately 88 years of the 100-year time horizon. Further, the IPCC assumes that at the end of its atmospheric lifetime, 25% of the CH4 is not oxidized to CO2 but rather is converted to formaldehyde and removed from the atmosphere through deposition on soils and water bodies. Therefore, it is assumed that only 75% of the carbon in CH4 becomes atmospheric CO2 through oxidation. To put simply, the GWP calculation consists of summing all radiative forcing of CH4 molecules over their lifetime, adding the radiative forcing of the 75% of the carbon that is oxidized to CO2 (over ~88 years rather than 100), and treating the remaining 25% of carbon converted to formaldehyde as having been removed from the atmosphere.
Figure 2: Highly simplified model representation of CH4 radiative forcing integrated over 100 years. Note: this graph does not accurately depict the decay curve of an annual methane pulse[4] over time, it depicts radiative forcing over time for CH4 released in year 0.
The 27.0 GWP value
The 27.0 GWP value, identified by the IPCC AR6 as non-fossil, biogenic, and fossil-combustion, is produced by decreasing the “solely methane” value (i.e., 27.9) to reflect the IPCC’s assumption that 25% of the CH4 at the end of its atmospheric lifetime is not oxidized to CO2 but rather is converted to formaldehyde and removed from the atmosphere through deposition. In other words, the emission of methane and subsequent oxidation of that methane is assumed to indirectly result in the subsequent sequestration of carbon. The result is that when applying this methane GWP value, an emission source is claiming to have removed a portion of CO2 (i.e., the 25% of carbon converted to formaldehyde) from the atmosphere for at least 100 years. The calculation of this GWP value also appears to assume that during the average 12 years of a methane molecule’s atmospheric lifetime, the radiative forcing of the molecule of CO2 is avoided before the carbon in the methane is returned to the atmosphere as carbon dioxide.
Figure 3: Highly simplified representation of CH4 radiative forcing integrated over 100 years. Note: this graph does not accurately depict the decay curve of an annual methane pulse[4] overtime, it depicts radiative forcing over time for CH4 released in year 0.
And then there is formaldehyde
Now before we can answer the question of which GWP value you should use in which situation, we must first introduce the complexity that formaldehyde adds.
The IPCC’s assumption is that some methane (i.e., 25%) is converted into formaldehyde—instead of being oxidized in the atmosphere to CO2—and is effectively removed from the atmosphere. The issue with this assumption is it only considers results from atmospheric chemistry models (Boucher et al., 2009). Yet, to fully understand the fate of this formaldehyde, we should also look to other fields of environmental science.
Formaldehyde (CH2O) in the atmosphere is subject to both dry and wet deposition processes onto soils, bodies of water, and other surfaces. Once deposited, it degrades through various environmental processes such as aqueous or surface chemical reactions or microbial activity. Its environmental persistence in each of these cases is expected to be brief (i.e., days), with the ultimate fate of the carbon atoms within it being predominantly oxidation to CO2 (Chénier, 2003).[6] In sum, we would expect much of the carbon in methane that is partially oxidized into formaldehyde to be fully oxidized to CO2 (and H2O) in a matter of days.
Chemistry lesson aside, what values should I use?
We will address each value separately and explain its appropriate uses.
When to use the 27.9 “solely methane” GWP value
The 27.9 “solely methane” GWP value is appropriate for application to emission source categories in which the CO2 from the oxidized CH4 is not a net addition of CO2 to the atmospheric carbon cycle. These categories include those involving carbon of biogenic (non-fossil) origin and those involving fossil fuel combustion. Biogenic source categories, such as biomass combustion or livestock enteric fermentation, again simply return carbon that was recently in the atmosphere CO2 back to the atmosphere. For fossil fuel combustion categories, the estimation of CO2 typically accounts for the addition of CO2 to the atmosphere from the oxidation of methane released from the same source (e.g., when the estimation of CO2 from fuel combustion assumes 100% oxidation of carbon in the fuel).[7] If the higher GWP value (i.e., 29.8) is applied to such fossil-combustion source category calculations instead, then the radiative forcing effect of the oxidized CO2 will be double counted—once in the estimation of combustion CO2 emissions and a second time in the calculation of GWP-weighted CH4 emissions that result from combustion.
When to use the 29.8 GWP value
The 29.8 GWP value is appropriate for application to emission source categories involving fugitive methane emissions from fossil fuel production and delivery systems (e.g., oil & gas systems, coal mining). It is also applicable to non-energy-related emission source categories for industrial processes when both of the following conditions are true:
- the carbon in the emitted methane is of fossil origin (e.g., carbide production, ethylene production) and
- the carbon in the process is not separately estimated to have been fully oxidized and emitted as carbon dioxide.
Do not use the 27.0 GWP value
We recommend that you do NOT use the 27.0 GWP value in GHG accounting.[8] The IPCC’s assumption that the deposition of formaldehyde removes CO2 from the atmosphere does not seem realistic for the purpose of GHG accounting. It seems unlikely to us that most of this carbon would be stored permanently in soils or other carbon pools. Rather, it is likely that much of the carbon in deposited formaldehyde is oxidized or biologically metabolized to CO2 and returned to the atmosphere due to formaldehyde’s relatively short-lived terrestrial and aquatic environmental persistence.
The AR6 GWP model used to quantify GWP values applies evidence-based biogeochemistry assumptions that are valid but present issues from a GHG accounting perspective. The limitations of the formaldehyde assumptions can have problematic implications when applied to a comprehensive quantification of fossil-derived CO2 emissions to the atmosphere across all source categories (i.e., for GHG accounting).
Summary recommendations
The recommendations below are intended to assist those engaged in GHG inventories and other GHG accounting work to select the most appropriate methane GWP value given the existing research limitations. Table 2 summarizes these recommendations. The “fossil – fugitive & process” labeled GWP should be used for fossil fuel fugitive emission sources (e.g., oil & gas systems, coal mining) and industrial processes where carbon in methane is of fossil origin (e.g., carbide production, ethylene production). All other sources of methane emissions should use the “fossil – combustion & non-fossil” GWP value.
Table 2: Recommended methane GWP values and labels based on AR6
GWP Descriptive Label | AR6 GWP value (100 yr) |
---|---|
Methane (fossil – fugitive & process) | 29.8 |
Methane (fossil – combustion & non fossil) | 27.9 |
Not recommended for use in GHG accounting applications | |
Further thoughts
First, and critically, you should recognize that the uncertainty on these methane GWP values is roughly ±40%, or ±11 GWP points. And so, the differences we are addressing here are small compared to the uncertainties. Still, as experts preparing GHG emission estimates, we are called upon to present point estimates that can be used for mitigation targets and/or compliance tracking purposes. So, while we always need to understand and be transparent about the uncertainties in our estimates, we still must choose a specific GWP value to apply, and that value should be the most scientifically appropriate.
Second, we argue that a methane GWP value of 30.65 (27.9 + 2.75 which is the or 44/16 = 2.75) might be more appropriate to be applied to fossil-fugitive & process emission sources rather than the 29.8 value (Boucher et al., 2009; Gillenwater, 2008). The higher value of 30.65 would not incorporate the questionable assumption that formaldehyde leads to permanent sequestration of carbon. It would also account for a full 100 years of radiative forcing from the oxidized CO2, which is consistent with the 27.9 value applied to fossil fuel combustion emission source categories. Hopefully, this issue can be addressed in the next IPCC assessment report.
Lastly, another option exists that would allow us to use a single methane GWP value for all emission source categories. It would be the “solely methane” 27.9 value. This option entails accounting for the radiative forcing from the atmospheric oxidation of CH4 to CO2 separately in our physical GHG accounting (Gillenwater, 2008). Methodological guidance on how to perform such estimates in GHG inventories is also provided in the IPCC Guidelines (see section 7.2.1.5 here).
Recognizing our recommendations deviate from current recommendations from most GHG reporting programs on the selection of methane GWP values, we strongly urge the next IPCC assessment report process to consider how GWPs are utilized in practice within physical GHG accounting and how computed model assumptions may inadvertently create problems for GHG accounting. Specifically, model assumptions can be better aligned with applied GHG accounting and target setting.
GWP Timeframe
We have focused on the application of a 100-year GWP timeframe (i.e., the length of time over which the radiative forcing from unit release of CH4 is integrated) as it is most commonly used in intergovernmental, regulatory, and voluntary contexts. However, in the specific case of methane, there is an ongoing debate over whether it is preferable to use a 20-year GWP timeframe in place of the 100-year one.[9] The primary purpose of GWPs is to make the emissions of different GHGs (on a mass basis) additive on a radiative forcing effect weighted basis. And, by definition, GWP is an index set relative to carbon dioxide, which has a much longer atmospheric lifetime than methane. Ultimately, the decision regarding which time frame to use across GHGs (e.g., 20 versus 100 years) is one based on policy and ethical considerations rather than science, as it involves balancing social considerations of intergenerational justice and climate risk tolerance as well as predictive beliefs on future mitigation costs. The 20-year time frame emphasizes more immediate climate action while deemphasizing long-term impacts of non-methane GHGs. In other words, the implication of choosing a 20-year time frame for all GHGs would be to exclude much of the longer-term radiative forcing effects of non-methane GHGs. There is no technically objective correct choice of GWP time frame. In fact, there is no prohibition in generating emissions inventory totals on total mass of CO2-e with both GWP timeframes for different analysis purposes.
Acknowledgments
We are thankful for the insightful comments from and discussions with Prof. Bill Collins (University of Reading), although any errors and all recommendations provided are solely those of the authors.
Recommended Citation
Gillenwater, M. and Benchimol, A. (2024). “Which methane GWP value do I use? And which value should not be used?” Seattle, WA. Greenhouse Gas Management Institute, October 2024. https://ghginstitute.org/2024/10/17/which-methane-gwp-value-do-i-use/
Cover image via Unsplash: Katie Rodriguez.
Footnotes
[1] Lifetime is a general term used for various time scales characterizing the rate of processes affecting the concentration of trace gases. The following lifetimes may be distinguished: Response time or adjustment time (Ta) Response time or adjustment time (Ta) is the time scale characterizing the decay of an instantaneous pulse input into the reservoir. The term adjustment time is also used to characterize the adjustment of the mass of a reservoir following a step change in the source strength. Half-life or decay constant is used to quantify a first-order exponential decay process. See Response time or adjustment time for a different definition pertinent to climate variations.
The term lifetime is sometimes used, for simplicity, as a surrogate for adjustment time. In simple cases, where the global removal of the compound is directly proportional to the total mass of the reservoir, the adjustment time equals the turnover time: T = Ta. An example is CFC-11, which is removed from the atmosphere only by photochemical processes in the stratosphere. In more complicated cases, where several reservoirs are involved or where the removal is not proportional to the total mass, the equality T = Ta no longer holds.
Carbon dioxide (CO2) is an extreme example. Its turnover time is only about 4 years because of the rapid exchange between the atmosphere and the ocean and terrestrial biota. However, a large part of that CO2 is returned to the atmosphere within a few years. The adjustment time of CO2 in the atmosphere is determined from the rates of removal of carbon by a range of processes with time scales from months to hundreds of thousands of years. As a result, 15 to 40% of an emitted CO2 pulse will remain in the atmosphere longer than 1,000 years, 10 to 25% will remain about ten thousand years, and the rest will be removed over several hundred thousand years. In the case of methane (CH4), the adjustment time is different from the turnover time because the removal is mainly through a chemical reaction with the hydroxyl radical (OH), the concentration of which itself depends on the CH4 concentration. Therefore, the CH4 removal rate S is not proportional to its total mass M.
Turnover time (T) (also called global atmospheric lifetime) is the ratio of the mass M of a reservoir (e.g., a gaseous compound in the atmosphere) and the total rate of removal S from the reservoir: T = M/S. For each removal process, separate turnover times can be defined. In soil carbon biology, this is referred to as mean residence time. (IPCC, 2021)
[2] Since the Second Assessment Report (SAR or AR2), IPCC has published 20-year, 100-year, and 500-year time horizon in their subsequent assessment reports. Historically, the overwhelming preference to use the 100-year time horizon has its roots in the Kyoto Protocol – which established the specific GWP time horizon and IPCC publication (i.e., AR2 or SAR) that countries were to use for mandatory reporting purposes.
[3] The instantaneous radiative forcing effect of a unit pulse of methane is integrated over 100 years to calculate a total radiative forcing.
[4] Pulse refers to the emissions that occur each year and the variability in year to year emissions.
[5] If the GWP was calculated using the full 100-year time horizon for the CO2 from oxidized methane the value would be 30.65, which is the 27.9 GWP value plus 2.75 (i.e., the stoichiometry ratio of kg CO2/kg CH4).
[6] For more on the environmental fate of formaldehyde see this draft U.S. EPA report: https://www.epa.gov/system/files/documents/2024-03/2.-formaldehyde-draft-re-chemistry-fate-and-transport-assessment-public-release-hero-march-2024.pdf
[7] In some cases, the same may be true for some industrial process categories that utilize fossil fuel feedstocks where methane and CO2 are emitted.
[8] Note, that many GHG reporting and crediting programs and guidance documents list this value without realizing the problematic underlying assumptions for GHG inventory and other GHG accounting applications.
[9] The IPCC has typically published GWP values over three standard timeframes—20, 100, and 500 years. The selection of these three options by the IPCC was reasonable but still arbitrary (i.e., it could have alternatively been 10, 50, and 300 years or any other set).
References
Boucher, O., Friedlingstein, P., Collins, B., Shine, K.P., 2009. The indirect global warming potential and global temperature change potential due to methane oxidation. Environ. Res. Lett. 4, 044007. https://doi.org/10.1088/1748-9326/4/4/044007
Chénier, R., 2003. An Ecological Risk Assessment of Formaldehyde. Human and Ecological Risk Assessment: An International Journal 9, 483–509. https://doi.org/10.1080/713609919
Gillenwater, M., 2008. Forgotten carbon: indirect CO2 in greenhouse gas emission inventories. Environmental Science & Policy 11, 195–203. https://ghginstitute.org/2010/07/13/what-is-different-about-methane-ch4-emissions-the-forgotten-co2-in-ghg-emissions-accounting/
Intergovernmental Panel on Climate Change (IPCC). (1996). Climate Change 1995: The Science of Climate Change. Contribution of Working Group I to the Second Assessment Report of the Intergovernmental Panel on Climate Change. Cambridge University Press.
Intergovernmental Panel on Climate Change (IPCC). (2021). Climate Change 2021: The Physical Science Basis. Contribution of Working Group I to the Sixth Assessment Report of the Intergovernmental Panel on Climate Change [Masson-Delmotte, V., P. Zhai, A. Pirani, S. L. Connors, C. Péan, S. Berger, N. Caud, Y. Chen, L. Goldfarb, M. I. Gomis, M. Huang, K. Leitzell, E. Lonnoy, J. B. R. Matthews, T. K. Maycock, T. Waterfield, O. Yelekçi, R. Yu, and B. Zhou (eds.)]. Cambridge University Press. doi:10.1017/9781009157896.
Intergovernmental Panel on Climate Change (IPCC). (2022). Climate Change 2022: Mitigation of Climate Change. Contribution of Working Group III to the Sixth Assessment Report of the Intergovernmental Panel on Climate Change [Shukla, P. R., J. Skea, R. Slade, A. Al Khourdajie, R. van Diemen, D. McCollum, M. Pathak, S. Some, P. Vyas, R. Fradera, M. Belkacemi, A. Hasija, G. Lisboa, S. Luz, and J. Malley (eds.)]. Cambridge University Press. doi:10.1017/9781009157926.
Why do you use the outdated 100-year horizon?
What matters are the next twenty years by all accounts, and there, the radiative forcing is more like 80. Everything else is painting a better picture than science tells you there is.
Michael S.,
We address this question (i.e., the selection of integration time frame) at the bottom of the post. One can argue that the 20 year time frame is more appropriate. But one can also make a strong argument that this neglects to consider the even more important long term climate impacts of CO2 and other GHGs. This is an ethical and policy judgment. Saying that the use of 20 year GWP values is the scientifically correct values to use is simply not justified by evidence.
Dear Michael, Alissa,
I would like to bring your attention to this article we have just published in Environmental Research Letters, which supports your arguments regarding formaldehyde and calculates new GWP100 values for methane accordingly.
https://iopscience.iop.org/article/10.1088/1748-9326/ad8edd
Hi Ivan,
Thank you for reaching out and sharing your recent article. Looking forward to reading your piece. Good to see our findings are aligned. Hoping AR7 addresses this accordingly. Cheers!